 | 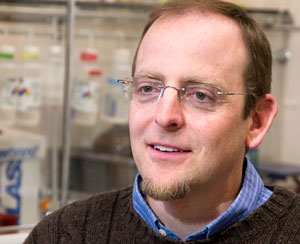 |
 |
| WHOI biochemist Ben Van Mooy is applying his interest in biofilms to the problem of biofouling, the accumulation of barnacles, algae, and other organisms on ship hulls. (Photo by Tom Kleindinst, Woods Hole Oceanographic Institution) |
 |
 |
 | 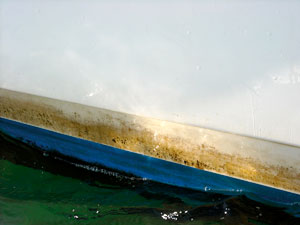 |
 |
|
Enlarge Image
Within a day of entering seawater, a ship hull acquires a thin coating of bacteria—the beginnings of a slimy biofilm that larger organisms such as algae and barnacles can attach to. The biofilm along the waterline of this boat is probably several weeks old. (Photo by Jim Canavan, Woods Hole Oceanographic Institution) |
 |
 | 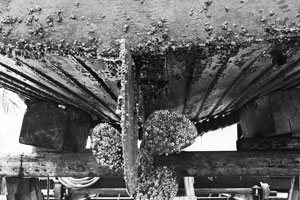 |
 |
|
Enlarge Image
Fouling organisms drive up operating expenses by increasing frictional drag on the hull and propellers. Fouling of the rudder can also interfere with the ship's ability to maneuver. This ship, photographed in 1943, was overdue for a session in drydock. (Robert H. Cole, Woods Hole Oceanographic Institution Archives) |
 |
 | 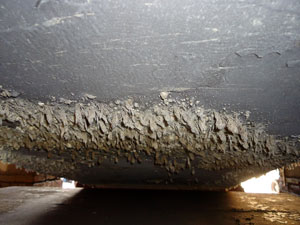 |
 |
|
Enlarge Image
As seen during its 2012 visit to drydock, the hull of R/VAtlantis carried patchy accumulations of barnacles and algae. WHOI ships are put into drydock twice in every five-year period for routine maintenance, including removal of fouling organisms. (Photo by Dutch Wegman, Woods Hole Oceanographic Institution) |
 |
 | 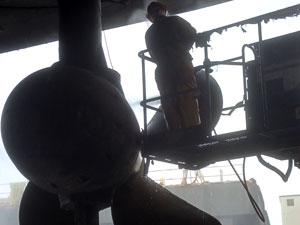 |
 |
|
Enlarge Image
A worker uses a high-pressure jet of water to clean organisms from the propeller of R/V Atlantis during the ship's period of drydocking in 2012. Removal of biofouling organisms can also occur while the ship is in the water, although access is more difficult. "Almost annually, the Knorr crew requests that we bring in divers and polish the propellers again," said WHOI Marine Engineer Theo Moniz. "They actually gain speed by that." (Photo by Dutch Wegman, Woods Hole Oceanographic Institution) |
 |
 | 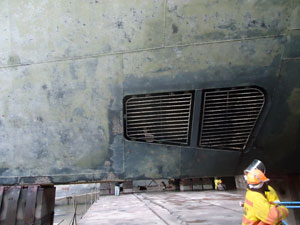 |
 |
|
Enlarge Image
Even internal parts of a ship suffer from biofouling. This grate on R/V Atlantis covers the inlet where seawater is drawn into pipes to cool the ship's mechanical systems. The grate keeps large organisms from entering the pipes, but small ones, including larval barnacles, can still enter, and the grate itself can develop a heavy encrustation of fouling organisms. This photo was taken while the ship was in drydock in November, 2012. The grate is low on the starboard bow; another one is on the port bow. (Photo by Dutch Wegman, Woods Hole Oceanographic Institution) |
 |
 | 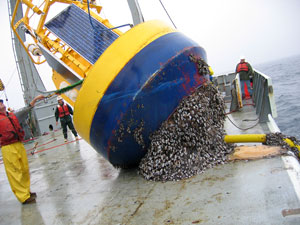 |
 |
|
Enlarge Image
Oceanographers fight a never-ending battle against fouling organisms that attach to their instruments, moorings, and buoys, as well as to ships. This Nootka buoy, which is designed to receive acoustic data signals from instruments on the seafloor, had just been recovered after being in the cool waters off Vancouver Island for about 13 months. (Photo by Norman Farr, Woods Hole Oceanographic Institution) |
 |
 | 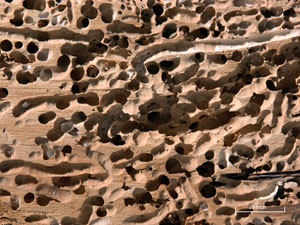 |
 |
|
Enlarge Image
This piece of wood is riddled with holes made by soft-bodied clams known as shipworms. Steel, the material of choice for ship hulls since the mid- to late 1800s, is worm-proof but vulnerable to fouling by algae, barnacles, and other organisms that attach to hard surfaces. (Photo by Tom Kleindinst, Woods Hole Oceanographic Institution) |
 |
 | 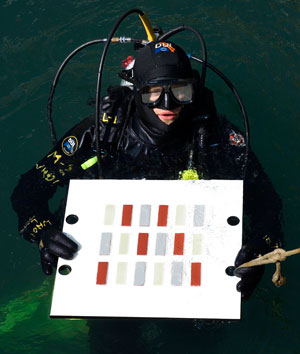 |
 |
|
Enlarge Image
In an earlier experiment by Van Mooy's lab, WHOI research associate Byron Pedler prepared to suspend a biofilm test plate just below the surface of the water in Vineyard Sound. Each test plate bore strips of hull material coated with an antifouling paint or a fouling-release paint, or left uncoated. Here, at the beginning of the experiment, all the strips were clean and free of biofilm. (Photo by Ben Van Mooy, Woods Hole Oceanographic Institution) |
 |
 | 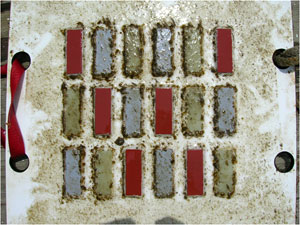 |
 |
|
Enlarge Image
After a short time in the water—a few weeks in winter, several days in summer—a test plate becomes covered with a biofilm, a slimy coating of bacteria and compounds they exude. Small algae (green strands) have attached to the biofilm on this plate. Note that the red strips, which were coated with a copper-based antifouling paint, are mostly free of biofilm. Both the green strips, which had no special coating, and the gray strips, which were coated with a fouling-release paint, are covered with biofilm. (Photo by Ben Van Mooy, Woods Hole Oceanographic Institution) |
 |
 | 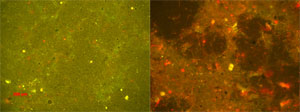 |
 |
|
Enlarge Image
Photomicrographs of ship hull biofilms from one of Van Mooy's tests. The yellow and red spots are microbial cells; the red spots indicate cells that contain chlorophyll, such as algae. The left panel shows a copper-based anti-fouling coating with just a few cells adhering to it. The right panel shows a fouling-release coating with abundant cells and the mucilaginous slime that they secreted. The difference in color between the two panels is due to the way light from the microscope interacted with the two coatings. (Photomicrographs by Ben Van Mooy, Woods Hole Oceanographic Institution) |
 |
 | 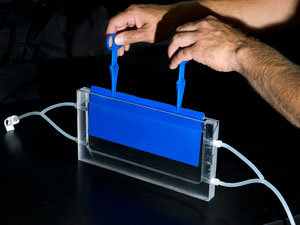 |
 |
|
Enlarge Image
WHOI research associate Justin Ossolinski built narrow tanks that allowed scientist Ben Van Mooy to bathe bacteria-covered plates in a small volume of continuously flowing seawater. The plates had been placed in Vineyard Sound until a robust biofilm of microbes developed. Then they were brought into the lab and placed in the tanks. By selectively filtering out specific kinds of cells, Van Mooy was able to determine the rates of cell settling, proliferation, detachment, and predation on the biofilm. (Photo by Tom Kleindinst, Woods Hole Oceanographic Institution) |
 |
 | 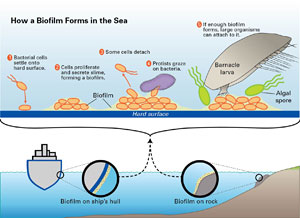 |
 |
|
Enlarge Image
Biofilms form when bacteria settle onto a hard surface (1), where they proliferate and produce slime (2). Most efforts to fight biofouling have targeted these steps. WHOI biochemist Ben Van Mooy thinks a better approach might be to enhance the processes that naturally reduce the size of biofilms: detachment of some of the bacteria (3) and predation by protists (4). (Illustration by Jack Cook, Wood Hole Oceanographic Institution) |
 |
 | Related Links |
 |
|
As long as sailors have been going down to the sea in ships, they have been coming back with their ships’ hulls coated with barnacles, algae, and slimy gunk.
And for just as long, mariners have searched for ways to repel and remove the adhesive organisms. Solutions ranged from scraping the hulls with heavy chains to more wacky approaches such as electrifying the water around vessels, coating the hulls with glass, and turning entire ships into magnets.
Yet “biofouling,” as it is called, continues to plague ocean-going vessels.
“For over two millenia, mariners have sought ways to prevent biofouling,” said Ben Van Mooy, a biochemist at Woods Hole Oceanographic Institution (WHOI). “That tells you some things. One is, it’s a really, really difficult problem.”
It’s also very, very expensive. A 2011 study estimated that biofouling increases the frictional drag on ships so much that it costs the U.S. Navy between $180 and $260 million per year in added fuel use.
Then there’s the cost of taking each ship out of service for a few weeks and putting it into dry dock so the hangers-on can be scraped off or dislodged with high-pressure jets of water; and the cost of preventive measures that enable ships to get by with a scraping only every two years, rather than every few months.
Add in other nations’ military ships, fishing and recreational boats, and thousands of commercial vessels, and the global costs of biofouling are astronomical.
To combat this age-old scourge, Van Mooy has sought to unravel the complex biological factors that set the stage for marine organisms to settle and attach on surfaces in the first place, and then to survive and thrive on them. With this new approach, he is revealing strategic points where people can intercede to curtail the biofouling process.
Tiny beginnings
The immense problem starts at the microscopic level—with a slippery layer of marine bacteria that larger organisms attach to (and sometimes eat).
“If you clean a ship or put a new ship to sea, it’s coated with microbes within a day,” said Van Mooy. “Not enough to see, but the first microbes are starting to lie down. And then the slime begins to grow over the course of weeks. That is essentially a gateway community to the barnacles and other things.”
“The slime” is a biofilm, a thin sheet of bacteria that stick to each other and to a matrix of molecules they exude to communicate with each other and to provide a hospitable environment for themselves. Once the slime forms, the rush is on, as algae and the larvae of creatures such as barnacles attach and begin to grow.
Routine scraping of an average size commercial vessel can yield up to 200 tons of organisms. And that’s just the fouling on the hulls. Of equal concern are the pipes, ranging up to about a foot in diameter, that move cold seawater throughout a ship’s heat-generating mechanical systems.
“We pump tons of seawater through piping on the ship,” said WHOI Port Engineer Dutch Wegman, who deals with biofouling on the institution’s research vessels. “You get fouling inside the piping, which reduces the flow. Then we might not be able to cool our engines well, and so we lose speed because we can’t go to full power. It can be a real problem.”
Fending off fouling
With the high costs of removing organisms from ships and the even higher costs of not removing them, inventors and ship operators have come up with hundreds of methods for reducing biofouling. Almost all of the would-be solutions have been variations on two themes: prevent organisms from sticking to the hull, or poison them if they do.
In the era of wooden hulls, the primary threat came not from barnacles that attached to the outside of ships, but from marine worms—actually, long, thin, soft-bodied clams—that tunneled through the wood. The Romans used lead sheeting to protect against shipworms, giving their ships an edge in commerce and in war. (It added substantial weight, but it was on the ships’ bottoms, contributing to the vessels’ stability.) Lead-covered hulls still hosted barnacles that had to be scraped, but at least the wood remained strong.
In the mid 1700s, the British began sheathing ship bottoms with copper, which repelled barnacles and other organisms and warded off wood-wasting worms.
“That was a radical technical advance,” said Van Mooy. “It was probably one of the things that contributed to the emergence of England as a naval superpower in the 18th century.”
For a few decades, copper sheathing was all the rage; but when steel hulls came into use in the 1800s, copper could no longer be employed because it hastened corrosion of the steel. Steel alone was impervious to worms but susceptible to slime, algae, and barnacles.
Once more, the hunt was on for a way to combat biofouling. The most popular solution, and the one still in use today: Paint.
Preventive paints
The success of copper sheathing prompted shipowners to try coating their hulls with paint containing copper. The idea was that small amounts of copper would leach into the water immediately next to the ship, poisoning any small creatures that approached. Several other toxic ingredients were also tried, including arsenic, mercury, strychnine, cyanide, and radioactive materials. A 1952 review of their performance by WHOI scientists indicated that some of the formulations worked well against barnacles. It did not mention what effects they had on the people who applied them.
Wegman recalled that many years ago, the WHOI ship Oceanus was among the first to use tin-based antifouling paints. It was so effective that the ship didn’t need to be scraped and repainted even five years after its initial coating with the paint. “Tin was wonderful,” he said. “But it’s a funeral of death—everywhere it went, it was killing off, to some extent, everything around it.”
So tin-based paints were banned. The U.S. Navy and WHOI now use the old standby, copper-based “antifouling” paint. But like tin paints, the very reason it works has become a matter of concern.
“The reason this paint is so good is this is a really, really, reallyeffective toxin,” said Van Mooy. “Now copper is being outlawed, because the ships come into port and then they slough off all this copper, and it contaminates the harbor. So the Navy’s got a problem.”
Slick solution
Recognizing the dangers of toxic coatings, paint developers have come up with slippery “fouling release” paints that defeat biofouling not by killing the organisms, but by making it impossible for them to stick to the ship. When a ship is in dock or moving slowly, microbes and larger organisms can attach to it; but when the ship moves quickly, the force of water moving past the hull knocks them off.
That’s an effective approach for ships, such as commercial vessels, that go fast and spend most of their time on the move. But it’s much less useful for those that spend a lot of time in port or holding still at one site.
“A lot of these ships that cruise at 25 to 30 knots love it,” said Wegman. “But with an oceanographic research ship, first of all, we’re slow going out”—top speed for WHOI vessels is 11 knots—“and then we stop at the study site. Some of our scientists’ favorite places to stop are these wonderfully productive places like the Sea of Cortez. Scientists love it, and we hate it, because the growth that goes on on our propellers and the piping inside the ship is significant. So the slippery stuff didn’t work for oceanography at all.” And it’s not a solution for the Navy, whose ships are in port about 80 percent of the time.
New targets
Van Mooy studies microbial ecology in the ocean , and since forming biofilms is a fundamental way of life for marine microbes, he has been interested in the process for several years. But he hadn’t made the connection to the fouling of ship hulls until the manager of the Navy’s program on biofouling research invited him to submit a research proposal to understand the process in more detail and identify steps that might be good targets for antifouling measures.
“I’m not really working on solving the problem, I’m working onunderstanding the problem,” Van Mooy said. “Materials scientists and paint designers are coming up with new technologies to prevent biofouling. But those aren’t going to work unless they know what processes to target with the new technologies..”
Biofilms grow when cells settle onto the surface and proliferate once they are attached. These are the steps that have gotten the most attention in fouling prevention efforts.
But microbial biofilms don’t just keep getting thicker and denser, said Van Mooy. Some of the bacteria detach from the biofilm and return to a free-floating existence, perhaps in search of a less-crowded surface to colonize. Many bacteria that do stay attached are harvested by grazing protists or other zooplankton.
“This is very basic information that, surprisingly, is still just completely unknown” in the context of ship fouling, said Van Mooy. And that raised intriguing possibilities: Could boosting detachment or predation prevent biofouling?
A four-step approach
To measure all four key aspects of biofilm production—settlement, attachment, detachment, and predation—Van Mooy used metal plates about the size of standard notebook paper. He left some parts of the plates bare and coated others with antifouling treatments. Then he attached the plates to pylons just below the sea surface in Vineyard Sound.
“I put them out in the environment and let real communities do their thing,” said Van Mooy. “Ninety percent of the research in this field is focused on doing culture work—you take a plate like this and you go into the lab and throw it in with your favorite bacteria”—lab-grown cells that are the microbial equivalents of white lab rats. That sort of experiment has little relationship to the actual situation faced by ships in the sea. He was determined to work with native microbes.
“People test their coatings against these model organisms, and they perform brilliantly,” said Van Mooy. “Then they put ‘em in the ocean, and BAM!, they’re terrible.”
He left the plates in the seawater long enough for a robust natural biofilm to develop. In winter, that took about two months and in summer, just a few days
Then he brought the slimy plates into the lab and put each one into narrow tanks made by technician Justin Ossolinski, each bathed by a continuous flow of 200 milliliters (a bit more than ¾ cup) of seawater. He then let the biofilms continue to grow for 24 hours, so he could observe them over one complete daily solar cycle.
Some tanks received water fresh from Vineyard Sound, which contained other bacteria (that could settle onto the plate) and protists (that could eat biofilm cells).
Other tanks received water that had been filtered to remove bacteria that might settle onto the plates and yet other tanks received water filtered to remove protists and thus eliminate predation on the bacteria. These experiments allowed Van Mooy to estimate how settlement, attachment, detachment, and predation contribute to biofilm accumulation—or, in some cases, hinder it.
Van Mooy was pleased to find that his approach verified that copper-containing paints don’t stop microbes from settling, but the microbes die soon after they attach to the plate. The experiments also showed that accumulation of bacteria on a "fouling release" coating, which is not toxic, was almost 100 times faster than on the copper paint. Bacteria settled, proliferated, and formed a healthy biofilm on the slick coating because the water flowing past it was not moving fast enough to dislodge them.
Zeroing in
Those results confirmed that his experimental setup worked, but the real payoff came from looking at the processes that had not been studied in detail before. Van Mooy found that in the summer, biofilms formed fast but then reached a plateau. “Even though you have a lot of growth and a lot of settlement, these rates are opposed by grazing and detachment,” he said. “So you get a net near-steady state.”
In winter, by contrast, “the rates are quite a bit lower, but there’s still some accumulation happening.” Grazing by zooplankton was so much lower in winter that the biofilm continued to grow, but slowly.
Most exciting of all was how big a factor predation was. In both seasons, about two-thirds of the loss of cells was due to detachment and one-third to predation.
“Until we had done this experiment, we really didn’t know how big the predation rate was,” said Van Mooy. “Some people thought it might be really small compared to detachment. What this experiment is telling me is that one way to develop a new coating may be to encourage grazing. Is there something you could put in paint that would attract the microscopic grazers to come and ‘mow the lawn’ for you?”
He’s pressing that suggestion on the biofouling community, nudging engineers and paint designers to consider new approaches to keep ships organism-free. “This is the pitch I keep telling these guys: ‘You can’t spend your whole life focusing on stopping settlement. You’ve abandoned fighting proliferation, because you don’t want toxic coatings. The detachment angle doesn’t seem to be panning out for naval ships. So you should start working on grazing.’ ”
A WHOI legacy
Van Mooy’s experiments extend a long tradition of biofouling research at WHOI. In the years leading up to and during World War II, the Navy gave WHOI scientists a wealth of funding to work on biofouling. It was the first federally-sponsored research program at WHOI and the first effort anywhere to integrate basic research on the physics, chemistry, and biology of biofouling and its prevention. After the war, the Navy commended WHOI scientists for greatly advancing scientific knowledge of the fouling process and antifouling measures and saving it millions of dollars.
Wegman, who has dealt with the practical aspects of biofouling throughout his 35-year WHOI career, only recently learned of Van Mooy’s work. He is especially intrigued by the possibility that tiny predators could become allies in the never-ending battle against fouling organisms.
“Isn’t that the joy of working at Woods Hole Oceanographic?” he said. “Here we are, banging our heads against that wall, and we’ve got this guy over here saying, ‘Why don’t we look at it from a different direction?’ ”
Posted: December 5, 2012
|